Hey guys. So now we're going to get into the part that makes this chapter really fun, and that's the mechanism. Alright? So just so you guys know, I'm going to teach this section more in-depth than your professor taught it for this chapter. Why is that? Well, because I realized that mechanisms are such a big part of organic chemistry that if we can learn them as early as possible, it's going to help you guys so much more later on. Okay? You don't want to be learning about mechanisms for the first time in chapter 7 or chapter 8. That would be organic suicide. Alright? Because you're going to be very confused, and you're going to have to pick up the pace a lot. So, what we want to do is we want to use the concepts of acids and bases to introduce what the mechanism is. Alright? So we're going to talk about this in 2 parts. The first part is I want to talk about the reactivity of atoms. Why do they even react? And the second part is I want to show you how they react with electron movement. So let's go ahead and begin. So just so you guys know, the currency of organic chemistry, and I've said this before, is stability. Okay? Stability. Every atom wants to become more stable. Every molecule wants to become more stable. Alright? And it turns out that stability and reactivity you hear these words all the time, actually have an inverse relationship. Inverse. What I mean by that is opposite. That means if stability goes up, reactivity is generally going to go down. If reactivity is up, that means stability goes down. They're opposites of each other. Okay? So, how can we tell if a molecule is reactive or a molecule is unreactive? Alright? Like I said, this is not a section that's usually covered in your textbook. I have just built this from years of teaching Orgo and realizing that, hey, there's actually ways that we can predict this. Okay? So there are actually 4 really common ways that we know that something's going to be reactive. You guys ready? And you actually know 3 of these 4 already. Okay? The first one is formal charges. You guys remember those? Okay? Formal charges mean that an atom is not at its ideal bonding preference. Right? It has too many or too few valence electrons. Okay? Remember the sticks and the dots. So, what that means is that it's going to try to do anything in its power to try to go back to its bonding preference. So that means it has a very big reason to want to react with something else. If it can get rid of some electrons, maybe it can go back to the state that it wants to be in. Does that make sense? So that's the first one. If you see a formal charge, you know that this is going to be reactive. The second one is net dipoles. Okay? So net dipoles have to do with the fact that I have asymmetrical dipoles that are not perfectly cancelling out, and what that means is that I'm going to have partial charges in different places. Another way that I could say this is partial charges. Okay? So remember that a partial charge is the result of a net dipole. If I have a net dipole going to the right, that means that I would have a partial negative on one side and a partial positive on the other. Okay? So what I'm basically doing here is I'm saying if you have formal charges, a full charge, that's very reactive. But even if you have a partial charge, that's also very reactive. So now let's talk about 2 other things. The 2 other things are pi bonds. Okay? What we're going to find out is that pi bonds remember that we went over this in the first chapter? Pi bonds are not as strong as single sigma bonds. Right? They're actually much weaker in terms of the amount of energy that's saved. So what that means is that pi bonds are a really good source of electrons. Okay? And they're going to be pretty easy to break. Remember, sigma bonds are almost impossible to break many times, but pi bonds, they're not as stable, so they're easier to break. So pi bonds are going to also be reactive. I know I said the word break a lot of times, but then finally the 4th one is something you don't know yet, and that is steric effects. Okay? Steric effects are something that we're going to talk about more when we talk about, basically, cycloalkanes and we're going to talk about a bunch of other stuff a little bit later. But an example of steric effect, I just want to tell you, would be, like, something like ring strain. So imagine that I have a 3-membered ring and these bond angles are very, very, very tight. Usually, these bond angles would want to be, like, a 109.5, but instead, they're like 60 degrees. That's an example of ring strain. That means that these bond angles are not stable because the fact that they're just not their ideal position. Alright? And that's also going to make molecules reactive. Now do you need to deal with this one right now? No. But I just want to be comprehensive and let you know those are like, the 4 major things that make organic molecules reactive. Cool? So what I want to do is move on to an exercise and I want you guys to look at these 8 different compounds and just go ahead and look at the first box. The first box is literally a checkbox. I want you to either check off that it is reactive or put an x on it if it is not reactive. So I want you to go through maybe a through d, the first four, and I want you guys to look at those first four and see if you can find any of those four reasons why it would be reactive. If you can't then that means it's not going to be reactive.
- 1. A Review of General Chemistry5h 5m
- Summary23m
- Intro to Organic Chemistry5m
- Atomic Structure16m
- Wave Function9m
- Molecular Orbitals17m
- Sigma and Pi Bonds9m
- Octet Rule12m
- Bonding Preferences12m
- Formal Charges6m
- Skeletal Structure14m
- Lewis Structure20m
- Condensed Structural Formula15m
- Degrees of Unsaturation15m
- Constitutional Isomers14m
- Resonance Structures46m
- Hybridization23m
- Molecular Geometry16m
- Electronegativity22m
- 2. Molecular Representations1h 14m
- 3. Acids and Bases2h 46m
- 4. Alkanes and Cycloalkanes4h 19m
- IUPAC Naming29m
- Alkyl Groups13m
- Naming Cycloalkanes10m
- Naming Bicyclic Compounds10m
- Naming Alkyl Halides7m
- Naming Alkenes3m
- Naming Alcohols8m
- Naming Amines15m
- Cis vs Trans21m
- Conformational Isomers13m
- Newman Projections14m
- Drawing Newman Projections16m
- Barrier To Rotation7m
- Ring Strain8m
- Axial vs Equatorial7m
- Cis vs Trans Conformations4m
- Equatorial Preference14m
- Chair Flip9m
- Calculating Energy Difference Between Chair Conformations17m
- A-Values17m
- Decalin7m
- 5. Chirality3h 39m
- Constitutional Isomers vs. Stereoisomers9m
- Chirality12m
- Test 1:Plane of Symmetry7m
- Test 2:Stereocenter Test17m
- R and S Configuration43m
- Enantiomers vs. Diastereomers13m
- Atropisomers9m
- Meso Compound12m
- Test 3:Disubstituted Cycloalkanes13m
- What is the Relationship Between Isomers?16m
- Fischer Projection10m
- R and S of Fischer Projections7m
- Optical Activity5m
- Enantiomeric Excess20m
- Calculations with Enantiomeric Percentages11m
- Non-Carbon Chiral Centers8m
- 6. Thermodynamics and Kinetics1h 22m
- 7. Substitution Reactions1h 48m
- 8. Elimination Reactions2h 30m
- 9. Alkenes and Alkynes2h 9m
- 10. Addition Reactions3h 18m
- Addition Reaction6m
- Markovnikov5m
- Hydrohalogenation6m
- Acid-Catalyzed Hydration17m
- Oxymercuration15m
- Hydroboration26m
- Hydrogenation6m
- Halogenation6m
- Halohydrin12m
- Carbene12m
- Epoxidation8m
- Epoxide Reactions9m
- Dihydroxylation8m
- Ozonolysis7m
- Ozonolysis Full Mechanism24m
- Oxidative Cleavage3m
- Alkyne Oxidative Cleavage6m
- Alkyne Hydrohalogenation3m
- Alkyne Halogenation2m
- Alkyne Hydration6m
- Alkyne Hydroboration2m
- 11. Radical Reactions1h 58m
- 12. Alcohols, Ethers, Epoxides and Thiols2h 42m
- Alcohol Nomenclature4m
- Naming Ethers6m
- Naming Epoxides18m
- Naming Thiols11m
- Alcohol Synthesis7m
- Leaving Group Conversions - Using HX11m
- Leaving Group Conversions - SOCl2 and PBr313m
- Leaving Group Conversions - Sulfonyl Chlorides7m
- Leaving Group Conversions Summary4m
- Williamson Ether Synthesis3m
- Making Ethers - Alkoxymercuration4m
- Making Ethers - Alcohol Condensation4m
- Making Ethers - Acid-Catalyzed Alkoxylation4m
- Making Ethers - Cumulative Practice10m
- Ether Cleavage8m
- Alcohol Protecting Groups3m
- t-Butyl Ether Protecting Groups5m
- Silyl Ether Protecting Groups10m
- Sharpless Epoxidation9m
- Thiol Reactions6m
- Sulfide Oxidation4m
- 13. Alcohols and Carbonyl Compounds2h 17m
- 14. Synthetic Techniques1h 26m
- 15. Analytical Techniques:IR, NMR, Mass Spect7h 3m
- Purpose of Analytical Techniques5m
- Infrared Spectroscopy16m
- Infrared Spectroscopy Table31m
- IR Spect:Drawing Spectra40m
- IR Spect:Extra Practice26m
- NMR Spectroscopy10m
- 1H NMR:Number of Signals26m
- 1H NMR:Q-Test26m
- 1H NMR:E/Z Diastereoisomerism8m
- H NMR Table24m
- 1H NMR:Spin-Splitting (N + 1) Rule22m
- 1H NMR:Spin-Splitting Simple Tree Diagrams11m
- 1H NMR:Spin-Splitting Complex Tree Diagrams12m
- 1H NMR:Spin-Splitting Patterns8m
- NMR Integration18m
- NMR Practice14m
- Carbon NMR4m
- Structure Determination without Mass Spect47m
- Mass Spectrometry12m
- Mass Spect:Fragmentation28m
- Mass Spect:Isotopes27m
- 16. Conjugated Systems6h 13m
- Conjugation Chemistry13m
- Stability of Conjugated Intermediates4m
- Allylic Halogenation12m
- Reactions at the Allylic Position39m
- Conjugated Hydrohalogenation (1,2 vs 1,4 addition)26m
- Diels-Alder Reaction9m
- Diels-Alder Forming Bridged Products11m
- Diels-Alder Retrosynthesis8m
- Molecular Orbital Theory9m
- Drawing Atomic Orbitals6m
- Drawing Molecular Orbitals17m
- HOMO LUMO4m
- Orbital Diagram:3-atoms- Allylic Ions13m
- Orbital Diagram:4-atoms- 1,3-butadiene11m
- Orbital Diagram:5-atoms- Allylic Ions10m
- Orbital Diagram:6-atoms- 1,3,5-hexatriene13m
- Orbital Diagram:Excited States4m
- Pericyclic Reaction10m
- Thermal Cycloaddition Reactions26m
- Photochemical Cycloaddition Reactions26m
- Thermal Electrocyclic Reactions14m
- Photochemical Electrocyclic Reactions10m
- Cumulative Electrocyclic Problems25m
- Sigmatropic Rearrangement17m
- Cope Rearrangement9m
- Claisen Rearrangement15m
- 17. Ultraviolet Spectroscopy51m
- 18. Aromaticity2h 34m
- 19. Reactions of Aromatics: EAS and Beyond5h 1m
- Electrophilic Aromatic Substitution9m
- Benzene Reactions11m
- EAS:Halogenation Mechanism6m
- EAS:Nitration Mechanism9m
- EAS:Friedel-Crafts Alkylation Mechanism6m
- EAS:Friedel-Crafts Acylation Mechanism5m
- EAS:Any Carbocation Mechanism7m
- Electron Withdrawing Groups22m
- EAS:Ortho vs. Para Positions4m
- Acylation of Aniline9m
- Limitations of Friedel-Crafts Alkyation19m
- Advantages of Friedel-Crafts Acylation6m
- Blocking Groups - Sulfonic Acid12m
- EAS:Synergistic and Competitive Groups13m
- Side-Chain Halogenation6m
- Side-Chain Oxidation4m
- Reactions at Benzylic Positions31m
- Birch Reduction10m
- EAS:Sequence Groups4m
- EAS:Retrosynthesis29m
- Diazo Replacement Reactions6m
- Diazo Sequence Groups5m
- Diazo Retrosynthesis13m
- Nucleophilic Aromatic Substitution28m
- Benzyne16m
- 20. Phenols55m
- 21. Aldehydes and Ketones: Nucleophilic Addition4h 56m
- Naming Aldehydes8m
- Naming Ketones7m
- Oxidizing and Reducing Agents9m
- Oxidation of Alcohols28m
- Ozonolysis7m
- DIBAL5m
- Alkyne Hydration9m
- Nucleophilic Addition8m
- Cyanohydrin11m
- Organometallics on Ketones19m
- Overview of Nucleophilic Addition of Solvents13m
- Hydrates6m
- Hemiacetal9m
- Acetal12m
- Acetal Protecting Group16m
- Thioacetal6m
- Imine vs Enamine15m
- Addition of Amine Derivatives5m
- Wolff Kishner Reduction7m
- Baeyer-Villiger Oxidation39m
- Acid Chloride to Ketone7m
- Nitrile to Ketone9m
- Wittig Reaction18m
- Ketone and Aldehyde Synthesis Reactions14m
- 22. Carboxylic Acid Derivatives: NAS2h 51m
- Carboxylic Acid Derivatives7m
- Naming Carboxylic Acids9m
- Diacid Nomenclature6m
- Naming Esters5m
- Naming Nitriles3m
- Acid Chloride Nomenclature5m
- Naming Anhydrides7m
- Naming Amides5m
- Nucleophilic Acyl Substitution18m
- Carboxylic Acid to Acid Chloride6m
- Fischer Esterification5m
- Acid-Catalyzed Ester Hydrolysis4m
- Saponification3m
- Transesterification5m
- Lactones, Lactams and Cyclization Reactions10m
- Carboxylation5m
- Decarboxylation Mechanism14m
- Review of Nitriles46m
- 23. The Chemistry of Thioesters, Phophate Ester and Phosphate Anhydrides1h 10m
- 24. Enolate Chemistry: Reactions at the Alpha-Carbon1h 53m
- Tautomerization9m
- Tautomers of Dicarbonyl Compounds6m
- Enolate4m
- Acid-Catalyzed Alpha-Halogentation4m
- Base-Catalyzed Alpha-Halogentation3m
- Haloform Reaction8m
- Hell-Volhard-Zelinski Reaction3m
- Overview of Alpha-Alkylations and Acylations5m
- Enolate Alkylation and Acylation12m
- Enamine Alkylation and Acylation16m
- Beta-Dicarbonyl Synthesis Pathway7m
- Acetoacetic Ester Synthesis13m
- Malonic Ester Synthesis15m
- 25. Condensation Chemistry2h 9m
- 26. Amines1h 43m
- 27. Heterocycles2h 0m
- Nomenclature of Heterocycles15m
- Acid-Base Properties of Nitrogen Heterocycles10m
- Reactions of Pyrrole, Furan, and Thiophene13m
- Directing Effects in Substituted Pyrroles, Furans, and Thiophenes16m
- Addition Reactions of Furan8m
- EAS Reactions of Pyridine17m
- SNAr Reactions of Pyridine18m
- Side-Chain Reactions of Substituted Pyridines20m
- 28. Carbohydrates5h 53m
- Monosaccharide20m
- Monosaccharides - D and L Isomerism9m
- Monosaccharides - Drawing Fischer Projections18m
- Monosaccharides - Common Structures6m
- Monosaccharides - Forming Cyclic Hemiacetals12m
- Monosaccharides - Cyclization18m
- Monosaccharides - Haworth Projections13m
- Mutarotation11m
- Epimerization9m
- Monosaccharides - Aldose-Ketose Rearrangement8m
- Monosaccharides - Alkylation10m
- Monosaccharides - Acylation7m
- Glycoside6m
- Monosaccharides - N-Glycosides18m
- Monosaccharides - Reduction (Alditols)12m
- Monosaccharides - Weak Oxidation (Aldonic Acid)7m
- Reducing Sugars23m
- Monosaccharides - Strong Oxidation (Aldaric Acid)11m
- Monosaccharides - Oxidative Cleavage27m
- Monosaccharides - Osazones10m
- Monosaccharides - Kiliani-Fischer23m
- Monosaccharides - Wohl Degradation12m
- Monosaccharides - Ruff Degradation12m
- Disaccharide30m
- Polysaccharide11m
- 29. Amino Acids3h 20m
- Proteins and Amino Acids19m
- L and D Amino Acids14m
- Polar Amino Acids14m
- Amino Acid Chart18m
- Acid-Base Properties of Amino Acids33m
- Isoelectric Point14m
- Amino Acid Synthesis: HVZ Method12m
- Synthesis of Amino Acids: Acetamidomalonic Ester Synthesis16m
- Synthesis of Amino Acids: N-Phthalimidomalonic Ester Synthesis13m
- Synthesis of Amino Acids: Strecker Synthesis13m
- Reactions of Amino Acids: Esterification7m
- Reactions of Amino Acids: Acylation3m
- Reactions of Amino Acids: Hydrogenolysis6m
- Reactions of Amino Acids: Ninhydrin Test11m
- 30. Peptides and Proteins2h 42m
- Peptides12m
- Primary Protein Structure4m
- Secondary Protein Structure17m
- Tertiary Protein Structure11m
- Disulfide Bonds17m
- Quaternary Protein Structure10m
- Summary of Protein Structure7m
- Intro to Peptide Sequencing2m
- Peptide Sequencing: Partial Hydrolysis25m
- Peptide Sequencing: Partial Hydrolysis with Cyanogen Bromide7m
- Peptide Sequencing: Edman Degradation28m
- Merrifield Solid-Phase Peptide Synthesis18m
- 32. Lipids 2h 50m
- 34. Nucleic Acids1h 32m
- 35. Transition Metals5h 33m
- Electron Configuration of Elements45m
- Coordination Complexes20m
- Ligands24m
- Electron Counting10m
- The 18 and 16 Electron Rule13m
- Cross-Coupling General Reactions40m
- Heck Reaction40m
- Stille Reaction13m
- Suzuki Reaction25m
- Sonogashira Coupling Reaction17m
- Fukuyama Coupling Reaction15m
- Kumada Coupling Reaction13m
- Negishi Coupling Reaction16m
- Buchwald-Hartwig Amination Reaction19m
- Eglinton Reaction17m
- 36. Synthetic Polymers1h 49m
- Introduction to Polymers6m
- Chain-Growth Polymers10m
- Radical Polymerization15m
- Cationic Polymerization8m
- Anionic Polymerization8m
- Polymer Stereochemistry3m
- Ziegler-Natta Polymerization4m
- Copolymers6m
- Step-Growth Polymers11m
- Step-Growth Polymers: Urethane6m
- Step-Growth Polymers: Polyurethane Mechanism10m
- Step-Growth Polymers: Epoxy Resin8m
- Polymers Structure and Properties8m
Reaction Mechanism - Online Tutor, Practice Problems & Exam Prep

Understanding the mechanisms of organic chemistry is crucial for predicting molecular reactivity. Key factors influencing reactivity include formal charges, net dipoles, pi bonds, and steric effects. Reactive species are categorized as nucleophiles (electron donors) or electrophiles (electron acceptors). Electron movement is depicted using curved arrows, indicating bond formation and breaking. Heterolytic cleavage results in ions, while homolytic cleavage produces radicals. Mastering these concepts enables students to effectively analyze and draw mechanisms, enhancing their grasp of organic reactions.
Reactivity
Did you know that not all molecules are reactive? Only certain types of molecules will want to react in a mechanism. Let’s dig a little deeper into this...
Stability and reactivity generally have an inverse relationship. If a molecule is unstable in some way, it will want to react! Here are the 4 signs we can look for that determine reactivity:
How to tell if a molecule will be reactive or not.
Video transcript

These reactive trends ARE in order of strength (i.e. a formal charge will typically be more reactive than a dipole).
Using those indicators, let's see if the following molecules are reactive:

Reactivity of Molecules

Reactivity of Molecules
Video transcript
Alright. So these next ones, it turns out that they're all going to be reactive, just in different ways. So this one had a negative charge. A negative charge indicates a formal charge, so it will be reactive. That's actually one of the more reactive types of properties. F has a net dipole going up, meaning that it's going to have a partial negative and a partial positive. So once again, that's going to be reactive. Is water reactive? Actually, it is because it has a net dipole. Any molecule with a net dipole is going to be reactive to some extent. So water is also reactive.
And then finally, this last one's a little bit crazy, like you don't see lithium a lot. We haven't dealt with lithium a whole lot, but if we just use your electronegativity trends, you know that carbon is over here, and lithium is, like, all the way over here. Which one's more electronegative, the carbon or the lithium? The carbon is much more electronegative. So I would draw my dipole going towards the carbon, which means that I have a partial negative here, a partial positive there. Does that make sense? So once again, this one's also going to be reactive because it has partial charges. Now we actually understand that these are the molecules that are going to want to react.
Again, we are literally just matching these molecules to the 4 patterns discussed above.
Nucleophiles vs. Electrophiles
How to tell if charged molecules will react as nucleophiles or electrophiles.
Video transcript
But now how are they going to react? That's the next question. Okay? What are they going to react as? And it turns out that we can basically categorize all reactive organic molecules, this is important, into two different subtypes. Okay? And those two subtypes are basically negatively charged species and positively charged species. Okay? So a negatively charged species, I know you've heard these words before but I'm just going to remind you of what they are. A negatively charged species is known as a nucleophile. Okay? And the way that I'm going to, for this course, abbreviate nucleophile is, I'm often going to write nu-. Okay? And that stands for the fact that I have a negatively charged atom. Okay? And instead or a negatively charged atom or a negatively charged part of a molecule. And the important thing here is I'm generalizing because it's going to react about the same way no matter what type of nucleophile it is. As long as it's a nucleophile, it's going to react in certain types of reactions. Alright? Then the positively charged ones are going to be known as electrophiles. Okay? Electrophiles, I'm going to go ahead and summarize them using e+ meaning that it's a positively charged substance. And I also do want to talk about these names a little bit. What does nucleophile mean in electrophile? Well, if it's negatively charged, that means that what it wants is positive in order to balance out. Right? So nucleophile literally means it's a nucleus lover or it's a proton lover. Okay? So a nucleophile is going to want positive charges like protons in order to balance. Same thing with an electrophile. An electrophile has a positive charge, so it's going to want electrons to bond with or to make to react with in order to balance off that positive with a negative. Alright? So that's the way we think about it, but if you just want, just think the nucleophile is the negative and the electrophile is the positive. Alright? So now we look at all of these reagents. Okay? I gave you eight. And what I want you guys to do is figure out which of these are going to react more as electrophiles and which of them are going to react more as nucleophiles. For a few of these, that's easy. For a few of these, we can automatically tell. Okay? So let me go ahead and tell you guys which ones are the easy ones to identify. Okay? B is easy to identify. E is easy to identify. And actually, D is easy to identify. Okay? So for these, already just without knowing the next rule I'm going to teach you, you should be able to tell me if these are going to react as nucleophiles or electrophiles.
Now that we know how to determine if molecules are reactive, we still don’t know HOW they will react! There are two major subtypes of reactivity that we’ll often use in Orgo 1 and 2:

Note that a molecule doesn’t require a negative charge to be a nucleophile, but it needs to have similar properties (i.e. a source of electrons).
That said, try to identify if the following three molecules are nucleophilic or electrophilic.
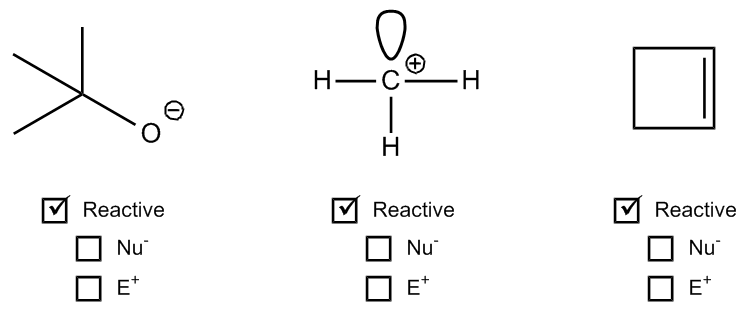
Nucleophile or Electrophile
Video transcript
Go ahead and start off with b. It has a positive charge, a full positive. So do you think it will be an electrophile or a nucleophile? Good job. I heard pretty much all of you say electrophile except that one annoying person that said nucleophile just to, like, annoy everyone off. I'm kidding. So no. Yeah, it was an electrophile because it's positively charged. In fact, what you could have done was just looked at the fact that I have an e positive and my carbon has a positive. Perfect. Okay? So now let's go to e. E is the next easiest one. So for e, I have a negative charge. What do you think that's going to be? Awesome. Wow. You guys are, like, on fire right now. That's going to be a nucleophile. Alright? Because it has a negative charge and nucleophiles tend to always have some kind of negative charge. Okay? Or some kind of negative region. Alright. So then what about d? Why is d easy? It doesn't have any charges. So how would I know if it's a nucleophile or an electrophile? And actually, the hint comes from what I said earlier about double bonds. I said that π bonds are a good source of electrons. Okay? This is just a general rule that I always want you guys to know. Double bonds are a really good source of electrons. So if it has a lot of electrons, what does that mean? Does that mean it's going to want more electrons or it's going to want protons? It would want protons, so that would make it a good nucleophile because it's going to want the nucleus or the proton. Okay? So basically, you see this double bond? What you think about is that you could almost think that it has a negative charge even though it doesn't. That's very wrong to draw. But I'm just having you imagine that those electrons are free lone pairs or whatever that could attack something else. Does that kind of make sense? Just think any time you see a double bond that it's a good source of electrons. So this is going to be a nucleophile. Alright?
How to tell if uncharged molecules will react as nucleophiles or electrophiles.
Video transcript
So now we just solved 3 out of 8. Out of these, 3 were really easy. Now, for the next ones, they're actually going to be tougher and we're going to need to know a new rule because, notice, all these other ones have both positive and negative regions. So, how do I know if it's a nucleophile or an electrophile? This is one of the biggest questions I receive in this section. "Johnny, how do I know that's a nucleophile? How do I know that's an electrophile?" It turns out that there's this rule we can use to figure out if it's going to react more like a nucleophile or more like an electrophile. Is that cool? So, I'm going to teach you that rule. And what that rule is this: the side of the dipole that has the highest bonding preference can be used to predict the nucleophilicity or electrophilicity. So, I agree with you. There are 2 charges on all these molecules. On the 5 molecules that are left, there's 2 charges: there's a positive and there's a negative. Now let's look at example a.
What I'm going to do is say which of the sides is the one that can make the most bonds? For example, the positive charge is on a carbon. How many bonds can carbon make? 4. The negative charge is on a chlorine. How many bonds can chlorine make? 1. Remember according to bonding preference. I told you, you're going to keep using bonding preferences all semester. So, is this going to react more like a nucleophile or more like an electrophile? The way that I look is I basically look at the 4, I look at the highest bonding preference, and I look at that charge. That's the one that I pay attention to because that's the one that has the highest bonding preference. So the answer is that this is actually going to be a really good electrophile. Why? Because the atom that has the highest bonding preference is the one that is positively charged. Isn't that cool? And that's the way you're going to use for all of these.
So, what we want you guys to do is we've already figured out b. What about c? Is c a good nucleophile or electrophile? I actually forgot to say this earlier. I should have pointed this out. Is c a nucleophile or an electrophile? This is actually one of the easy ones too. I forgot. So there were actually 4 easy ones and 4 hard ones. C is easy. So, I'm going to highlight this one as well. The answer is that it is none of them because I just told you it's not even reactive at all. So if it's not reactive at all, how can it be a proton lover or an electron lover? It doesn't even love anything; it just loves itself. It's a narcissist. So, it's just going to be inert. It's not going to react with anything, so I wouldn't consider it an electrophile or a nucleophile. Alright? So, that means that this has become a lot easier for you guys. All you need to do is identify f, g, and h, and tell me what you guys think in terms of if they're going to be electrophiles or nucleophiles. So go ahead and pause the video now and use that rule to determine these last 3.
So that wasn’t so hard, but those were the easy cases. What if you have nucleophilic AND electrophilic regions on the same molecule? Is it possible to determine how it will react? Yes it is!
Rule: The side of the dipole with the highest bonding preference (the atom that wants to make the most bonds) will determine how the molecule reacts.
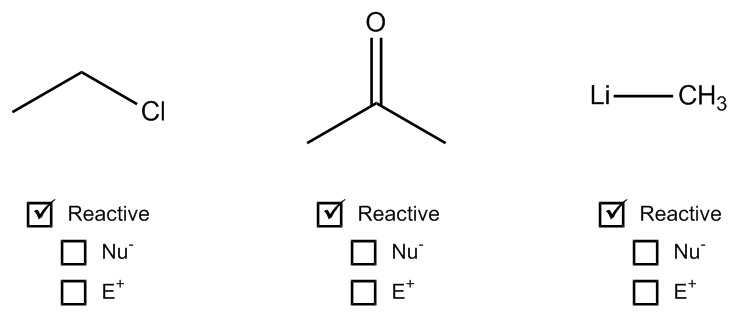
Nucleophile or Electrophile
Video transcript
Alright. So let's go ahead and start off with F. I have 2 charges. I look at the side with the highest bonding preference. Carbon can make 4 bonds. Oxygen can make 2 bonds. Which one is the one with the higher bonding preference? Oxygen. So, I'm going to look at that charge. So, you should have picked that that one is also going to be a very strong electrophile. Just so you guys know, I put these molecules here for a reason. A happens to be the most important electrophile of Organic Chemistry 1 and F happens to be the most important electrophile of Organic Chemistry 2. If you just know these 2 electrophiles, you're going to be, like, set for Organic Chemistry 1 and 2. The reason is because in Organic Chemistry 1 we're talking all about alkyl halides, what they do. And then in Organic Chemistry 2 we talk about carbonyl chemistry. We spend entire, like, 4 or 5 chapters just talking about electrophiles. So just a little preview. Now let's go on to H.
For H, I had lithium. Lithium can make how many bonds? Only 1. It's like hydrogen. Hydrogen is happy with 1 bond because it's in that first column. Okay? Then Carbon, once again, likes to have 4. So which one has the higher bonding preference? Carbon. So, I only look at this charge. That's a negative charge. That means this is going to be a nucleophile. Isn't that cool? So this is actually a molecule called an organometallic. Sounds so scary, right? Organometallic. What it just means is you have an organo or an organic part, which is the carbon, and you have a metal which is lithium. Remember lithium is a metal. So, organometallics are actually really common nucleophiles. And later on in this course, we're going to be using these all the time as really important nucleophiles. Cool? Awesome.
So, I've got the last one and I just want to tell you guys really quickly, water is an exception. Water can act as both. Okay? Because water, as you guys know, it has a dipole. Okay? But, I mean, basically what it has is it has these negative electrons, so it can accept electrons. Okay? I mean, it can give away electrons, but it can also accept electrons. Water is kind of neutral. So what that means is that water is going to be that one compound that's always going to be able to react as either a nucleophile or an electrophile depending on what the other substance is. Okay? So, I just want to say that water is kind of an exception. You can't tell if it's going to be a nucleophile or an electrophile until you see the other reagent and see how that one's reacting with it. Okay? Cool.
So with that said, I hope you guys are understanding why molecules react and how, in general terms, nucleophiles and electrophiles behave. And now, what I want to do in the next part is to talk about actually drawing out what these reactions look like. So let's go ahead and go for it.
Bond Making
Now we understand which molecules will want to react, and we are getting better at determining If they are nucleophiles or electrophiles, but how to they actually attack other molecules?
Reactive molecules share electrons to become more stable. Arrows are used to show which direction they are going.
- Arrows always move from regions of high electron density to low electron density
- By that logic, nucleophiles must always attack electrophiles.
- Each attacking arrow represents two electrons being shared.
- After the reaction is complete, replace that arrow with a new σ -bond
Summary:Molecules with lots of electrons will attack (draw an arrow to) molecules with a positive charge. Let’s get drawing!
Learning the rules of electron movement
Video transcript
So that first part was pretty fun, right? We just learned how to tell the difference between a nonreactive molecule, which is just inert, or a reactive molecule, which is actually going to try to make bonds with other molecules. Okay? On top of that, we also learned what type of reactivity they have. If they're going to react more like an electrophile or a nucleophile. Well, now what I want to do is actually teach you guys how to start drawing out these mechanisms. So what that means is we're going to start drawing electron movement and you guys are going to be probably the first people in your class to draw as good mechanisms as we're going to do now, but I'm not concerned. I know you guys are smart, and I think you guys are going to like this part. So let's go ahead and get started.
Reactive molecules are the ones that are going to try to do something about their reactivity. So what that means is if you're a nonreactive molecule, you're inert. You don't care. But if you're reactive, you're going to try to share electrons with another molecule in order to become more stable. Remember that we talked about that in the first chapter, how we form molecular orbitals in order to reach a lower energy state. Okay? So, it turns out that we're going to use curved arrows to indicate the direction that these electrons are going. And in organic chemistry, that's actually a huge part of this field. A huge part of this field is just figuring out where these electrons are moving. They start here, and they move over here, and then what do they do, and then they move over here. Those are called mechanisms. Okay? So, I'm just going to tell you guys right now, a huge part of this course is going to be drawing arrows and figuring out where these electrons are moving from atom to atom to atom. Okay?
So I just want to teach you guys or remind you guys a few things about how electrons move, and it turns out that these rules that I'm going to teach you are the same that we learned for resonance structures. Why? Because it turns out that resonance structures are the same thing in terms of that we're moving electrons from one atom to another. So let's go ahead and get started. So, remember that arrows are always going to move from regions of high electron density to low electron density. Okay? So what that means is that we always want to start from the part of the molecule that has the most electrons and move to a part of the molecule that doesn't have a whole lot. This is the same thing that we did in resonance structures. Okay? But back when we talked about resonance structures, we didn't know about nucleophiles and electrophiles. So now that I've taught you what a nucleophile is and what an electrophile is, which one do you think is always going to be the one that starts off the arrow? Because remember, we always start from the high area. Okay. So do we start off from the nucleophile or from the electrophile? And the answer is that we're going to start off from the nucleophile. Okay? Because the nucleophile is the one with the negative charge. Right? So we're always going to have nucleophiles that attack electrophiles. Okay? And a huge part of organic chemistry can be summed up as saying nucleophiles attack electrophiles. Honestly, like when it comes down to it, you could probably explain 50% of the reactions just talking like that. Okay? So it’s a very important point. Okay?
Another thing is that each attacking arrow is going to represent two electrons that are being shared. So every time that I draw one of these arrows, that means that I'm sharing two electrons with another atom. And what that means is that after the reaction has taken place, I'm going to replace whatever that arrow was. I'm going to replace it with a new sigma bond. Why is that? Because remember that a sigma bond equals two electrons being shared. Okay. So what that means is that an arrow is really just a fancy way of drawing a sigma bond in motion. That means I'm creating a new sigma bond. Does that make sense so far? I know it's a lot of words. So let's just go ahead and get started. So what I want you guys to do for these reactions is I know that you don't even know what these molecules are really, but we do understand nucleophiles and we do understand electrophiles. And we also understand how electrons move now. We kind of know. So what I want us to do first of all is determine the initial direction of electron movement by drawing the very first arrow of each mechanism. Even though we don't know what's going on here, it's okay because you can just follow the general rule of nucleophile and electrophile.
Using those rules, let's draw the mechanisms for the following reactions
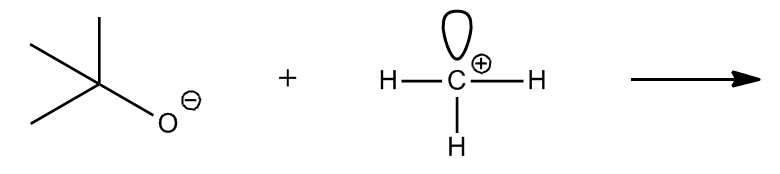
Drawing Electron Movement
Video transcript
In this case, I have a negative charge and I have a positive charge. Where do you think my arrow is going to start from? My arrow is going to start from the negative charge because remember that we always start from the nucleophile or the area of high density. So, what that means is that my arrow starts from the negative. Where do you think it's going to go to? Well, it's going to go to the area of least electrons, so that means that it's going to actually try to attack that carbon right there. Because what's going on is that the negative has too many electrons and it's saying, "Hey, I want to go ahead and link up to a positive charge in order to neutralize and to balance out." Does that make sense? So the first arrow of my mechanism is negative to positive. Does that make sense so far? That's very similar to what we did in resonance structures except now I have a full negative and a full positive. Okay?
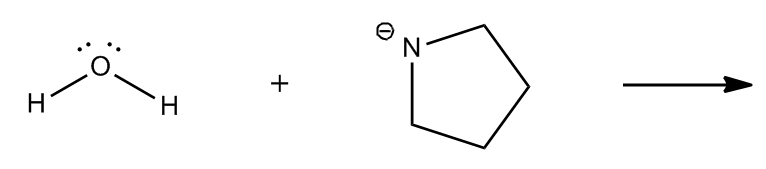
Drawing Electron Movement
Video transcript
For this next one, I also have a negative charge but I don't have a positive charge. So can you guys tell me where you think the arrow is going to start from? The arrow should start from the negative charge. That means it should start from the end. Okay? Now the question is where is it going to attack? In the first one, it was really easy. My electrophile was really easy to figure out because it just had a positive charge, but in this case, I have water. Now remember that water is an exception where it can act as either a nucleophile or as an electrophile depending on what it's reacting with. So where do you think it would attack here? I'm going to give you a hint. The only way to know that is by drawing out dipoles. So what I want you to do is draw out the dipoles on this molecule and figure out where the partial positive charge is. Once you've figured that out, then you can draw the rest of this arrow. So go ahead and pause the video, try to find the partial positive, and then draw the arrow directly attacking that positive. So go for it. Alright. So let's draw the dipoles. I had these dipoles from the lone pairs. I also had these dipoles pulling up towards the O. What that means is that the O has a partial negative because it has these electrons moving up towards it and then both of these H's are partial positive. Does that make sense? Because they're getting electrons pulled away from them. So if I were to complete this arrow, where do you think it would attack? What it's going to attack is one of the H's because the H is the thing that has the most positive charge on the entire molecule. Does that make sense? So now the H is what's acting as my electrophile. Isn't that interesting?

Drawing Electron Movement
Video transcript
Now let's go on to C. So for C, I don't have any charges. So now this is getting harder and harder. Okay? Where do you think I could react on C? Where do you think I would want to start from? I'm going to want to start from the thing that has the most electron density, the highest electron density. So, is there a nucleophile here that we learned from the previous page? Yes, there is. Remember that I told you guys that double bonds are good nucleophiles. Okay? So what that means is that even though I don't have a charge, I can still say that my arrow is going to start off from the double bond. Why? Because the double bond is a good source of electrons for a reaction. Now all I need to do is figure out which atom on the HCl it's going to react with. Okay? Is it going to react with the H or is it going to react with the Cl? The only way to know that for right now is to draw the dipole. So let's go ahead and draw the dipole. Which one is more electronegative? Chlorine. So let's go ahead and do that. That means I have a partial negative, a partial positive. Good. Okay. So now where is that negative charge going to want to attack? It's going to attack the H. Do you guys see that? Because once again, the H is the thing that has the positive charge and you can consider that the double bond pretty much has a negative charge because it has those free electrons that are very easy to donate. Does that make sense so far? I hope so. I hope that what you're seeing is that we're just always following a trend of nucleophile attacking the electrophile. Sometimes the electrophile is really obvious like in problem A, but sometimes we have to draw dipoles in order to know what the electrophile is. Cool so far? Awesome.
Bond Breaking
So now we know how to make bonds. Do we ever have to break bonds? How do we know if we do or we don’t?
Why we need to break bonds sometimes.
Video transcript
What I want to talk about is what we just did, which I call bond making. All of this involved forms of bond making where I am basically indicating that all these arrows represent the sharing of 2 electrons. And I said that after the reaction, you're going to replace that arrow with a sigma bond. So that means that all of these arrows that I just drew are eventually going to become little sticks that I draw off each of these atoms. So that's going to be a stick. That's going to be a stick. And that's going to be a stick. I hope that you understand that what I'm saying is that an arrow is just a really long way of drawing a single bond that's going to be formed.
Now what I want to talk about is bond breaking. Bond breaking is sometimes required in mechanisms, but it's only required when it's needed to preserve octets. So what that means is that just like resonance structures, remember that sometimes we would want to make a bond, but that bond would break the octet of a certain atom. So then what would we do to fix that? We would also break a bond. We would make a bond and then we would break a bond in order to preserve the octet of that atom. In the same way, we're going to want to break bonds in order to preserve octets of atoms that are getting attacked.
Bond breaking is sometimes required in mechanisms, but only when it is necessary to preserve octets.
The two ways to break bonds.
Video transcript
There are actually two ways to break bonds. I just want to teach you guys this really quickly. There's heterolytic cleavage, which means that you get ions. You get, like, a cation on one side and an anion on the other. Heterolytic, where hetero means different, just implies that you get different amounts of electrons on both. Notice that, for heterolytic cleavage, I use a full arrow.
The other option is homolytic cleavage. Homolytic cleavage means that each of the atoms, once the bond breaks, gets the same amount of electrons, so each of them gets a radical. Homolytic cleavage is done with half arrows. Notice that the head of that arrow is a little bit smaller. It's only big on one side. I know my head is right in the way of radicals, but you are going to see radicals.
What I want you guys to know is that, for the most part, in this entire chapter, we’re really just going to be dealing with heterolytic cleavage. We are not going to get into homolytic cleavage until we talk about radical chemistry, which is coming up in a few chapters, so you don’t have to worry about it yet. Cool?
Out of these two different ways, we will stick to heterolytic cleavage for the foreseeable future (we won’t discuss radicals for a few more chapters).
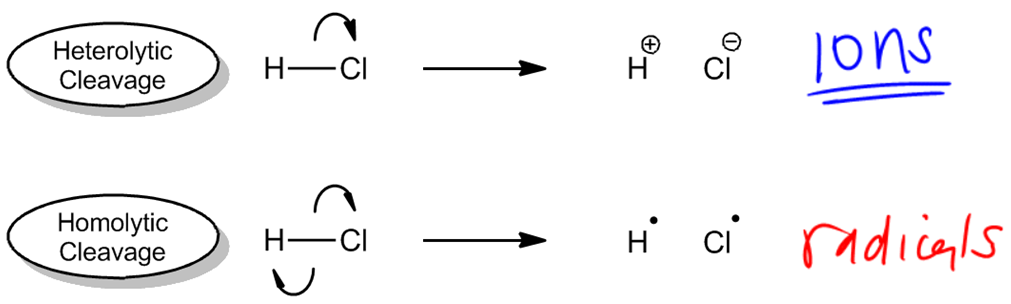
Identify if the following reactions require bonds to be broken. Draw the products.
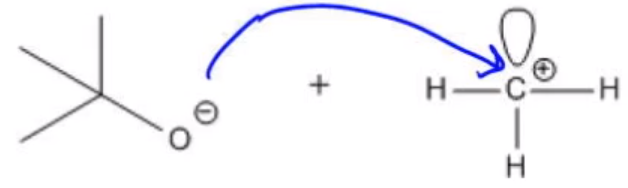
Identifying Bond Breaking
Video transcript
So what I want you guys to do now is we already talked about which bonds would be made. What I want you guys to do now is figure out which bonds would also have to be broken in order to not violate octets. Basically, I want you guys to finish these mechanisms on your own. Okay? I know that sounds rough, but let's go ahead and look at a. Okay? So for a, in my first step, I went ahead and I made a bond to this carbon. Okay? Now that I made a bond to that carbon, am I violating its octet? The answer is no. I'm not because check it out. This carbon had how many—sorry, had how many electrons before? 2, 4, 6. How many total electrons can carbon have? 8. 8. Okay? So if I add 2 more, is that going to break the octet? No. So that means that in this mechanism there's going to be no bond breaking. That mechanism is over. So my final product would look like this, O. Okay? So I just redrew the original. Now for the where that original where that curved arrow was, now I'm just going to draw a single bond. That single bond represents the 2 electrons that are being shared, and now I'm going to draw that connected to what was on the other side. So that’s going to be attached to a C with 3 H's. Isn't that interesting? That's my final product. So basically all I did was I made a bond but then I never violated the octet, so that's my final product. Okay? And once again, that new blue bond comes from the arrow. Okay? The arrow turned into a bond.
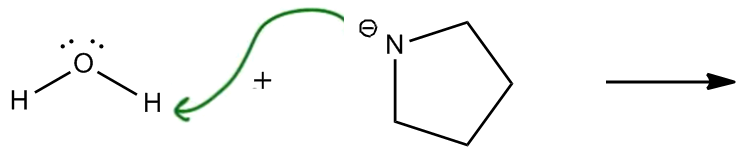
Identifying Bond Breaking
Video transcript
Now let's look at this next one. Okay? So I'll probably just have you guys do the last one on your own. Okay? So for n, notice that n went ahead and it grabbed the h right here. So now I have to ask you guys, how many bonds does hydrogen like to make? Remember, bonding preference. What does it like to make? Only one bond. Right? Well, check it out. This hydrogen already has a bond to the oxygen. Now what I'm doing is I'm making another bond to the nitrogen. That's my second bond. So is that hydrogen going to be happy making 2 bonds? No. Is it going to satisfy the octet? No. Remember that hydrogen wants to have 2 electrons in its octet. If I make 2 bonds to it, it will actually have 4 electrons in its octet. That sucks. So in order to make this bond, what must I do? I have to break a bond. Okay? So what that means is that I'm going to delete these dipoles because we don't need them anymore. We don't need those anymore. Okay? In order to make that bond, I'm going to have to break a bond. And the way that I break a bond is I just take 2 electrons from the bond and I give them to the o. So if I make a bond, I break a bond. Okay? And what that means is that now my final products are going to look like this. I'm going to get an h with an o, but now that o, instead of having 2 lone pairs, it has 3 because it has the 2 old lone pairs that it used to have. But now it has that extra lone pair that came from the single bond. Does that make sense so far? Cool. Then finally I have plus my ring structure with the n, but now notice that the n made a new bond to that h. So I'm just going to draw that now there's a stick attached to the h. Does that make sense? Where now that h came from what was attached to the o. Now the only thing we have to do is we have to add formal charges. So are there any formal charges here? Yeah. It turns out that o has too many electrons, so o would have a negative charge. Okay? And then the n already has a lone pair. It did from the beginning. So the n is perfect. The n likes its situation. Okay? So notice that in this reaction I actually exchanged a hydrogen and a negative charge. This is actually an acid base reaction, but you guys just drew out the entire mechanism yourselves. So you guys, like, honestly deserve, like, a pat on the back. Or you can give me a pat on the back if you want. Alright? Hopefully that wasn't too confusing. We're just doing the same thing over and over again. We're doing the first step is nucleophile to electrophile. The second step is to break if you need to.
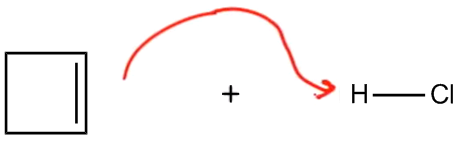
Identifying Bond Breaking
Video transcript
Alright guys. So the answer was that you do need to break this bond. The reason is because hydrogen only wants to have one bond, not two. It already has a bond to the chlorine. This arrow is going to be the second bond. That's not good for hydrogen. So, in order to make this bond, I have to break a bond. I'm going to take these two electrons and give them to the chlorine. Okay? So, what that means is that at the end, I'm now going to have a square that doesn't have a double bond anymore. Why? Because the two electrons from that double bond are what became the red arrow. Okay? Then I'm going to have a stick. That stick is the red arrow, the electrons that were shared, and that stick is going to be attached to a hydrogen. Okay? Awesome. So now that stick is attached to a hydrogen, and now I just have to figure out what's left. Well, I have a chlorine that used to have how many lone pairs? It used to have three. Okay? So, it used to have three lone pairs. Now, how many does it have? Well, it still has those three lone pairs, but then it also gained a lone pair from that bond. So this is four. So now that chlorine is going to have a negative charge. Does that make sense? Because it has 8 electrons instead of 7. Now, we're almost done, but the problem is that I mean, we're pretty much done. But the problem is that we have one more thing we have to watch out for, which is that anytime you break a double bond, you're breaking the amount of electrons that two atoms had. So this atom up here, this carbon, had an H and this carbon had an H. Are you guys cool with that so far? Now that it reacted, the top carbon still has that blue H, but now it has a new red H attached to it. The red H came from the HCl. Okay? So basically, what I'm saying is this H is the original one from here, and then this H is now the new one that came from there. Okay? But the bottom one still only has one H because it never attacked another H. So, what that means is that the bottom is now going to get a positive charge because now carbon wants to have four bonds, but it only has three. Okay? So this is actually your final product for this step. Okay? And it turns out that this is actually going to be a reaction that we explore a lot more in future chapters, but I'm just helping you guys to see how we can use nucleophiles and electrophiles to figure these things out even if you don't know the reaction. Okay? And it also turns out that if you have a good understanding of nucleophiles and electrophiles, even if you forget reactions in organic chemistry, you can still figure them out because you know these things. Alright?
Do you want more practice?
More setsHere’s what students ask on this topic:
What are the key factors that influence the reactivity of organic molecules?
The reactivity of organic molecules is influenced by several key factors: formal charges, net dipoles, pi bonds, and steric effects. Formal charges indicate that an atom is not at its ideal bonding preference, making it more reactive. Net dipoles create partial charges within a molecule, leading to reactivity. Pi bonds are weaker than sigma bonds and are easier to break, making them a good source of electrons. Steric effects, such as ring strain in cycloalkanes, also contribute to reactivity by creating unstable bond angles. Understanding these factors helps predict how and why molecules react.

How do you differentiate between nucleophiles and electrophiles in organic reactions?
Nucleophiles are negatively charged species or electron-rich molecules that seek positively charged centers, such as protons. They are often abbreviated as Nu-. Electrophiles, on the other hand, are positively charged species or electron-deficient molecules that seek electrons. They are often abbreviated as E+. To differentiate, look at the charges: nucleophiles have negative charges or lone pairs of electrons, while electrophiles have positive charges or electron-deficient areas. This distinction is crucial for predicting the direction of electron movement in reactions.

What is the significance of curved arrows in reaction mechanisms?
Curved arrows in reaction mechanisms indicate the movement of electrons during chemical reactions. They always start from a region of high electron density (nucleophile) and point towards a region of low electron density (electrophile). Each arrow represents the movement of two electrons, leading to the formation or breaking of bonds. Understanding how to draw and interpret these arrows is essential for visualizing and predicting the steps of a reaction mechanism, making it a fundamental skill in organic chemistry.

What is the difference between heterolytic and homolytic cleavage?
Heterolytic cleavage occurs when a bond breaks and both electrons are taken by one of the atoms, resulting in the formation of ions (a cation and an anion). This type of cleavage is represented by a full arrow. Homolytic cleavage, on the other hand, occurs when a bond breaks and each atom takes one electron, resulting in the formation of radicals. This type of cleavage is represented by half arrows. In most organic chemistry mechanisms, heterolytic cleavage is more common, while homolytic cleavage is typically discussed in the context of radical chemistry.

How do you determine if a molecule will act as a nucleophile or an electrophile?
To determine if a molecule will act as a nucleophile or an electrophile, examine the charges and bonding preferences. Nucleophiles are electron-rich and often have negative charges or lone pairs of electrons. Electrophiles are electron-deficient and often have positive charges. Additionally, consider the side of the dipole with the highest bonding preference. The atom with the highest bonding preference and a positive charge will likely act as an electrophile, while the atom with a negative charge will act as a nucleophile. This approach helps predict the behavior of molecules in reactions.

Your Organic Chemistry tutors
- Each of these compounds can react as an electrophile. In each case, use curved arrows to show how the electrop...
- In each reaction, label the reactants as Lewis acids (electrophiles) or Lewis bases (nucleophiles). Use curve...
- In each reaction, label the reactants as Lewis acids (electrophiles) or Lewis bases (nucleophiles). Use curve...
- Each of these compounds can react as a nucleophile. In each case, use curved arrows to show how the nucleophil...
- Each of these compounds can react as an electrophile. In each case, use curved arrows to show how the electrop...
- Label the reactants in these acid–base reactions as Lewis acids (electrophiles) or Lewis bases (nucleophiles)....
- Label the reactants in these acid–base reactions as Lewis acids (electrophiles) or Lewis bases (nucleophiles)....
- Label the reactants in these acid–base reactions as Lewis acids (electrophiles) or Lewis bases (nucleophiles)....
- Identify the arrow types that are shown in each of these arrow-pushing mechanisms. (iv)
- Show an arrow-pushing mechanism that forms the product on the right from the reactant at left. Only one arrow ...
- (••) Suggest an arrow-pushing mechanism for each of the following acid–base reactions. (c)
- Would you expect the following species to be electrophiles or nucleophiles? Some may be both. Explain your ans...
- Would you expect the following species to be electrophiles or nucleophiles? Some may be both. Explain your ans...
- Identify the nucleophile and the electrophile in each of the following reactions. (b)
- Show an arrow-pushing mechanism that forms the product on the right from the reactant at left. Only one arrow ...
- Show an arrow-pushing mechanism that forms the product on the right from the reactant at left. Two arrows are ...
- Show an arrow-pushing mechanism that forms the product on the right from the reactant at left. Here, three arr...
- Identify the arrow types that are shown in each of these arrow-pushing mechanisms. (iii)
- Acid–base reactions are reversible. Show a mechanism for the reverse of the reactions in Assessment 4.18.
- Identify the nucleophile and the electrophile in each of the following reactions.(a) <IMAGE>
- Show an arrow-pushing mechanism that forms the product on the right from the reactant at left. Two arrows are ...
- Identify the arrows shown by type and predict the product that should result.(e) <IMAGE>
- Identify the arrows shown by type and predict the product that should result.(a) <IMAGE>
- Would you expect the following species to be electrophiles or nucleophiles? Some may be both. Explain your ans...
- Using curved arrows, show the mechanism of the following reaction:
- For each of the reactions in Problem 15, indicate which reactant is the nucleophile and which is the electroph...
- Use curved arrows to show the movement of electrons in the following reaction steps a. b.
- We discuss the following reactions in subsequent chapters. Given the mechanisms shown, draw the mechanism of t...
- We discuss the following reactions in subsequent chapters. Given the mechanisms shown, draw the mechanism of t...
- a. Use curved arrows to show the flow of electrons that occurs in each step of the following mechanism
- Identify the electrophile and the nucleophile in each of the following reaction steps. Then draw curved arrows...
- The following reaction steps are shown using conventional electron pushing. (b) Use the bouncing arrow formali...
- Predict the product that results from the following 'pushed electrons.' (a) →
- Predict the product that results from the following 'pushed electrons.' (c) →
- Predict the product that results from the following 'pushed electrons.' (f) →
- Predict the product that results from the following 'pushed electrons.' (i) →
- The following reaction steps are shown using conventional electron pushing. (a) Draw the second product whose ...
- Why are NH3 and CH3NH2 no longer nucleophiles when they are protonated?
- In each case, circle the stronger nucleophile. (d) vs.
- (••) LOOKING AHEAD We show in Chapter 20 that α , β-unsaturated ketones are electrophilic at C₄ . Rationalize ...
- In each case, circle the stronger nucleophile. (c) vs.
- Provide a reasonable arrow-pushing mechanism for the following Lewis acid–Lewis base reactions.(a) <IMAGE&g...
- Provide an arrow-pushing mechanism for the following hypothetical base half-reactions. [These are only intende...
- (••••) THINKING AHEAD BCl₃ has an empty p orbital, so it is a strong Lewis acid. Would you expect an amide to...
- In each case, circle the stronger nucleophile.(b) <IMAGE> vs. <IMAGE>
- Based on the analysis you used in Assessment 17.3, which carbonyl would you expect to react most quickly with ...
- Classify the following nucleophiles as strong, weak, or intermediate. Would you expect each to add to a carbon...
- Would you expect a ketone or an ester to be more reactive with a strong nucleophile? Justify your answer.<I...
- Rank the reactivity of the following carbonyls with nucleophiles, from least reactive to most reactive.<IMA...
- The following reaction steps are shown using conventional electron pushing. (a) Draw the second product whose ...
- The following reaction steps are shown using conventional electron pushing. (b) Use the bouncing arrow formali...
- (••) For the following acid–base pairs, (v) show a mechanism for the reaction; (e) <IMAGE>
- Identify the electrophile and the nucleophile in each of the following reaction steps and then draw curved arr...
- Identify the electrophile and the nucleophile in each of the following reaction steps and then draw curved arr...
- Identify the electrophile and the nucleophile in each of the following reaction steps and then draw curved arr...
- Label the reactants in these acid–base reactions as Lewis acids (electrophiles) or Lewis bases (nucleophiles)....
- In each reaction, label the reactants as Lewis acids (electrophiles) or Lewis bases (nucleophiles).Use curved ...
- Each of these compounds can react as a nucleophile. In each case, use curved arrows to show how the nucleophil...
- Each of these compounds can react as a nucleophile. In each case, use curved arrows to show how the nucleophil...
- Which atoms in the enol ether would you expect to react with Br⁺ ? ...
- To which atom of formaldehyde would you expect H⁺ to add?
- For practice in recognizing mechanisms, classify each reaction according to the type of mechanism you expect: ...
- For practice in recognizing mechanisms, classify each reaction according to the type of mechanism you expect: ...
- For practice in recognizing mechanisms, classify each reaction according to the type of mechanism you expect: ...
- For practice in recognizing mechanisms, classify each reaction according to the type of mechanism you expect: ...
- Classify each reaction as a substitution, an elimination, or neither. Identify the leaving group in each react...
- Classify each reaction as a substitution, an elimination, or neither. Identify the leaving group in each react...
- Propose a mechanism for the rearrangement that converts an a-hydroxyimine to an a-aminoketone in the presence ...
- Classify each reaction as a substitution, an elimination, or neither. Identify the leaving group in each react...
- (••••) FROM THE LITERATURE The product of the Stille coupling reaction (A) tautomerizes in a basic solution to...
- Aluminum trichloride (AlCl3) dissolves in ether with the evolution of a large amount of heat. (In fact, this ...